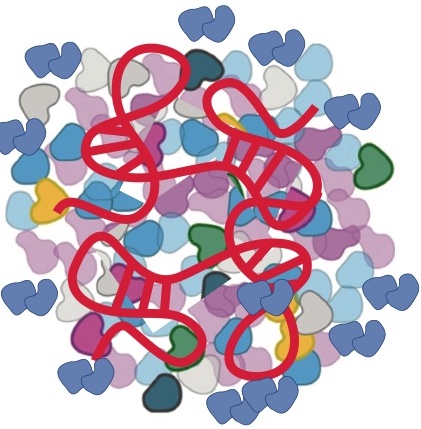
X-Chromosome Regulation
We often examine the epigenetic changes underlying cell fate transitions through the lens of the X-chromosome. Specifically, we elucidate the regulatory mechanisms governing gene expression on the X chromosome in female mammalian cells, which carries ~1000 genes in mouse and humans. By investigating these intricate processes, we aim to gain comprehensive insights into dosage compensation, a vital mechanism that ensures balanced gene expression between XX females and XY males. Understanding how X-linked gene expression is controlled in females provides crucial insights into the biological differences between men and women, has significant implications for the pathogenesis of X-linked disorders, and the female bias observed for various diseases, and provides ideas for the development of targeted therapeutic strategies. Overall, our work on the X chromosome deepens our understanding of the fundamental principles of gene regulation and epigenetics and their impact on human health.
Specifically, we study X-chromosome inactivation and X-chromosome dampening, two processes that are regulated by the long non-coding RNA XIST, and how XIST additionally controls the regulation of autosomal genes.
X-chromosome inactivation (XCI)
Our lab is dedicated to studying the molecular mechanisms behind X-chromosome inactivation (XCI). This process results in the silencing of genes on one of the two X chromosomes in female placental mammals, equalizing their gene expression dose between females with two X chromosomes and males with one X chromosome. The master regulator of XCI is Xist (X-inactive specific transcript), a 17kb long noncoding RNA (lncRNA), that itself is encoded on the X chromosome. The XCI process was first described more than 60 years ago and the Xist was identified in 1990. Since then, it has remained elusive how Xist RNA can spread along an entire chromosome and mediate silencing. Our lab made tremendous progress towards understanding these questions. Specifically, we aim to decipher how Xist becomes expressed appropriately on the X chromosome, how Xist RNA localizes along the entire X chromosome, how the RNA interacts with protein complexes, and how, through these interactions, Xist RNA controls chromosome conformation, heterochromatin formation, and gene silencing across the X chromosome, and how genes on the X chromosome escape from the XCI process.
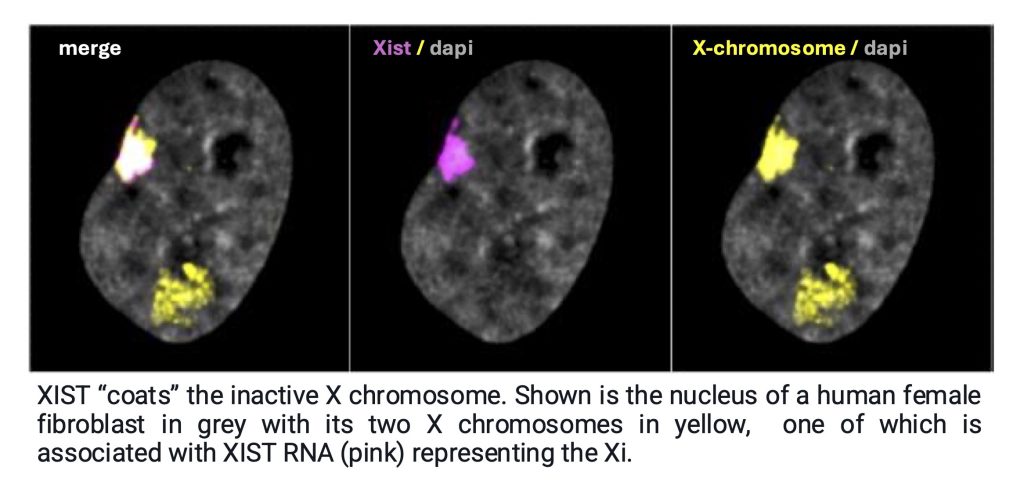
Our work on Xist has been crucial not only for understanding the regulation of the X chromosome but also for shedding light on the broader role of lncRNAs. Mammalian genomes encode thousands of lncRNAs, many of which localize to the nucleus, suggesting they play significant roles in the regulation of gene expression. By studying Xist RNA, we can gain insights into the general principles of how lncRNAs interact with chromatin and influence nuclear architecture and gene expression.
One of our initial findings was that the repressive polycomb complex PRC2 and H3K27me3 accumulate on the inactive X chromosome (also called the Xi) in an Xist RNA-dependent manner (Plath et al, Science, 2003). As result, H3K27me3 has become synonymous with X-inactivation and is the most widely used marker of the inactive X in and outside the field. This finding has also led to the model that long-noncoding RNAs can recruit chromatin-modifying complexes to their target sites.
We created the first model to explain how Xist-RNA spreads along the X-chromosome, which identified a unique role of 3D-genome organization in lncRNA function. In collaboration with the Guttman lab at Caltech, we explored Xist RNA localization at high resolution using the RAP-seq method. We discovered that Xist spreads from its site of transcription on the X chromosome to spatially proximal, but linearly distal, regions of the X chromosome (Engreitz et al, Science, 2013). Thus, pre-existing long-range chromatin interactions of the genomic Xist locus dictate Xist’s spread across the X chromosome. Other lncRNAs may similarly take advantage of chromosome conformation to identify target sites in close spatial proximity, which could even reside on other chromosomes. A proximity-guided search strategy might explain how low-abundance lncRNAs can reliably identify their genomic targets.
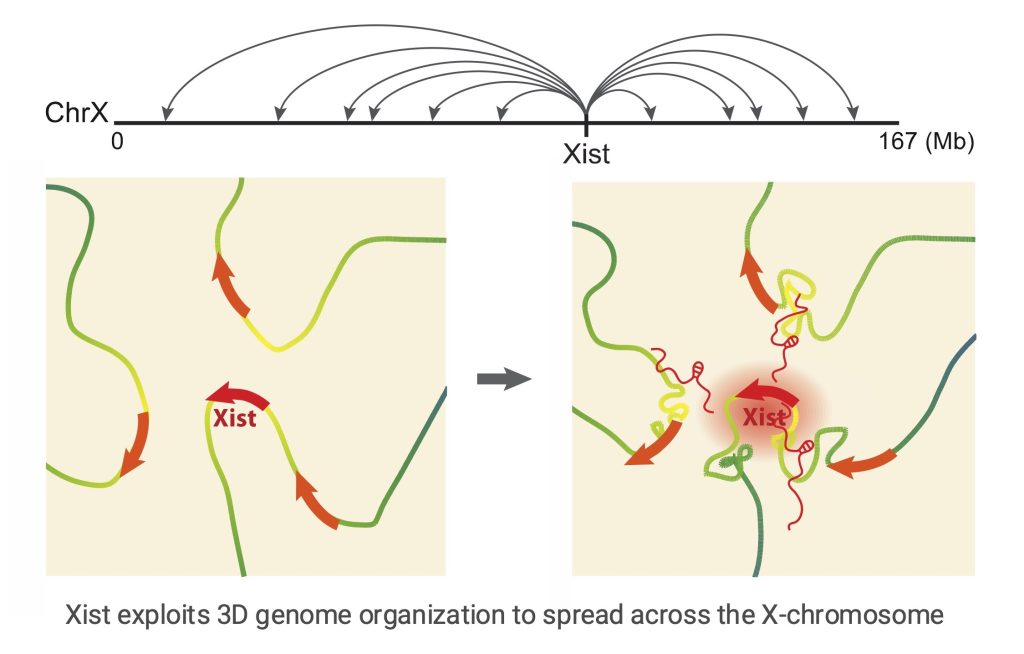
Our findings show that protein condensation is critical for Xist’s function. By characterizing how Xist utilizes its interacting proteins to form a silent compartment across the entire X chromosome, we discovered that the Xist RNA concentrates its interacting proteins and induces their condensation within the Xi. We made two critical findings:
Xist is an unusually long mature RNA, close to 17kb in length. It harbors six repetitive elements termed A – F. Through deletion analysis of these repeat elements, we first identified the E-repeat of Xist as crucial for maintaining the localization of Xist to the Xi territory and gene silencing across the X chromosome (Pandya-Jones et al, Nature, 2020). We showed that RNA-binding-proteins PTBP1, MATRIN3, TDP43, and CELF1 assemble on the E-repeat-element of Xist (Pandya-Jones et al, Nature, 2020). These proteins are well-characterized RNA-processing factors. However, we found that they can form higher-order assemblies, when concentrated by Xist RNAs which contains multivalent protein binding sites for these proteins. When bound to Xist, these factors engage in self-aggregation and heterotypic interactions that induce the formation of a higher-order Xist–protein network within the inactive X-chromosome territory. We found that deletion of these proteins (or of the E-repeat) does not disrupt the initial Xist RNA spread or XCI initiation but interferes with the maintenance of both gene silencing and Xist sequestration within the X-territory. Overall, our work revealed an unanticipated mechanism for the maintenance of gene silencing and Xist localization, a new epigenetic memory mechanism, and gives us a unique handle for novel approaches for the reactivation of the inactive X chromosome (Pandya-Jones et al, Nature, 2020).
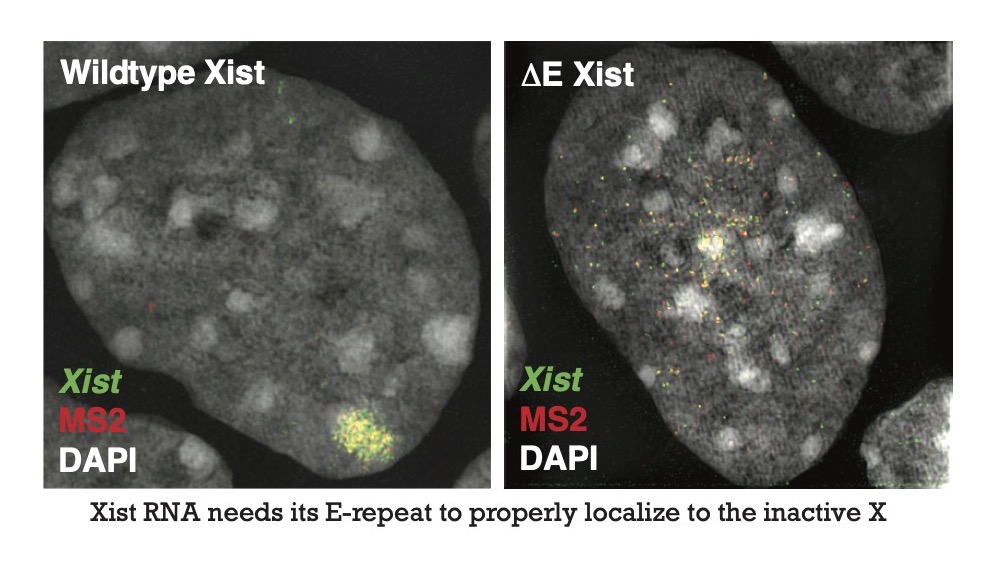
Our E-repeat study made us think about protein condensation in the context of XCI. For decades, based on conventional fluorescence microscopy, Xist RNA has been seen as a cloud covering the inactive X chromosome, leading to the model that Xist-protein complexes associate with the regulatory elements of every X-linked gene to achieve silencing. However, super-resolution microscopy showed that Xist distributes as diffraction-limited foci on the inactive X. In our second major study, we discovered that the Xist ‘cloud’ consists of ~50 foci, each containing two Xist molecules, indicating that ~100 RNA molecules within ~50 defined structures orchestrate X-chromosome inactivation (Markaki et al, Cell, 2021). Live-cell super resolution imaging showed Xist foci are locally confined to chromatin and do not move throughout the X. This raised the question of how this small number of locally confined Xist foci and molecules can ensure the silencing of the ~1000 X-linked genes across the 167Mb of the X chromosome.
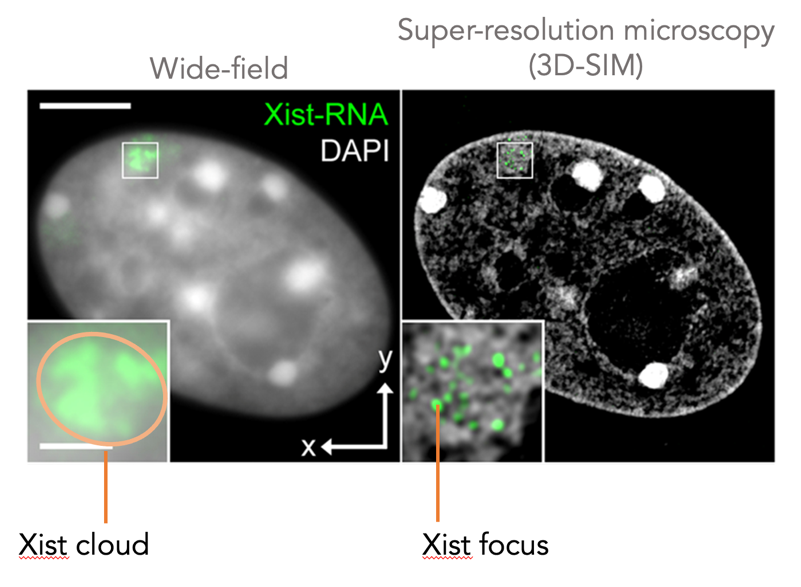
To address this question, we analyzed the distribution and behavior of Xist-interacting proteins. Intriguingly, we found that they form nano-sized multi-protein assemblies around each Xist focus. We refer to these Xist-seeded proteinaceous nanostructures as Xist-associated supra-molecular complexes (SMACs). We suggest that thousands of protein molecules surround the two Xist molecules in each SMAC. Thereby, Xist RNA forms a stable core of SMACs that anchors proteins, which can bind to and dissociate from Xist-SMACs with fast kinetics. Intrinsically disordered domains of Xist-interacting proteins are required for their high accumulation around Xist foci. Intriguingly, we also found that without this protein accumulation (by interrupting IDR interactions) silencing of X-linked genes don’t not occur. Thus, we propose that by establishing high local concentrations of transiently binding effector proteins through SMACs, Xist induces gradients of proteins allowing them to act at genomic locations on the X without continuous association with the Xist RNA. We also uncovered that the high concentration of polycomb protein incorporation into SMACs progressively induces higher-order chromatin changes and compaction, gradually repositioning all regions of the X chromosome into the territory of Xist foci and enabling SMACs to deterministically repress genes across the entire X over time.
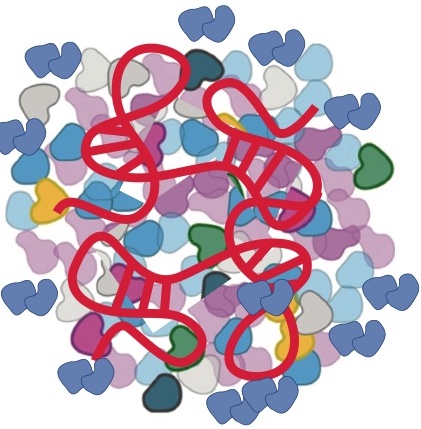
Overall, our work on Xist defines protein crowding as the mechanism of action by which few Xist molecules can silence the genes on the X chromosome and therefore demonstrates how a sub-stoichiometric amount of Xist can induce the silencing of a much larger number of genes. There has been considerable debate about how lncRNAs can robustly regulate gene expression, given that they are often expressed at low levels; our work provides a model that is generally applicable for chromatin-associated lncRNAs involved in the regulation of gene expression and therefore has broad implications beyond Xist.
Regulation of the X chromosome in humans
Originally, our studies of Xist were performed in the mouse system. Since a distinct X-chromosome dosage compensation mechanism exists in early human development, we are now also studying the regulation of the X chromosome in female human cells. This is giving us unique insights into human-specific features of female development.
X-chromosome dampening (XCD)
In contrast to mice, where imprinted XCI occurs during pre-implantation development and random XCI in the embryo, female human pre-implantation embryos do not display XCI. Instead, both X-chromosomes are active but express their genes at a reduced transcriptional output, which is coined X-chromosome dampening (XCD). Interestingly, XIST is expressed from both active yet dampened X chromosomes. Therefore, in human pre-implantation embryos XIST expression is uncoupled from complete gene silencing as seen in the XCI process. We uncovered that female germ cells when they are specified also have XIST expression and exhibit XCD, similar to the human female pre-implantation embryo (Chitiashvili et al, Nature Cell Biology, 2020).
These findings raised the question of whether XCD is mediated by XIST. The paucity of pre-implantation embryos or human germ cells for research makes a thorough mechanistic understanding of XCD difficult. To overcome this issue, we explored whether human pluripotent stem cells can capture the XCD state. Pluripotent stem cells exist in the naïve and primed states, which correspond to the pre-implantation and post-implantation epiblast, respectively. We explored if various published culture conditions for naïve human pluripotent stem cells can capture the XCD state and showed that specific naive culture formulations are compatible with the XCD state (Sahakyan et al, Cell Stem Cell, 2016). These findings set the stage for determining how XCD is regulated and whether XIST plays a role in this process. Using various genomics and functional approaches, we found that XIST spreads across the X chromosome and mediates XCD in female naïve human pluripotent stem cells utilizing many of the mechanisms that XIST exploits in the XCI process (Dror et al, Cell, 2024). Thus, XIST can induce XCD and XCI in human development. How it is determined when XIST mediates XCD versus XCI occurs remains a topic for future exploration (Dror, Tan et al Current Opinion, 2024).
XIST can also function in trans – on autosomes
In cells with XCD, i.e. in female naïve human pluripotent stem cells, human primordial germ cells and human pre-implantation embryos, XIST is in a non-typical, dispersed configuration wherein XIST foci are detected throughout a large portion of the nucleus. The significance of this dispersed configuration was unknown.
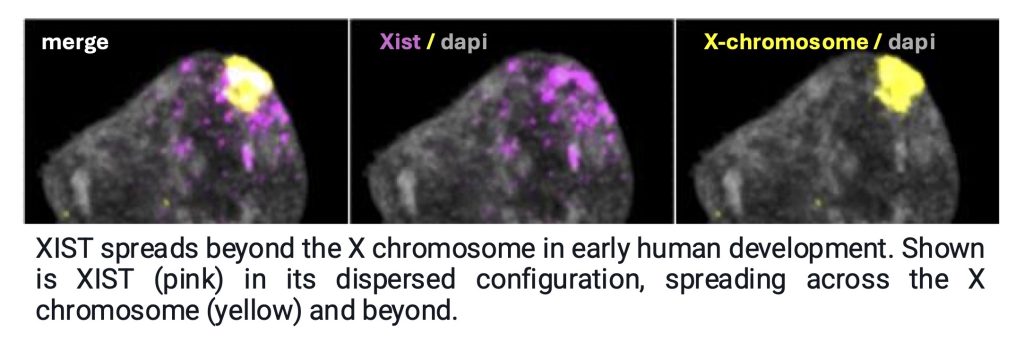
Surprisingly, we found that in addition to the X chromosome, XIST also targets specific autosomal regions, when it is in its dispersed configuration in human female naïve pluripotent stem cells. Like on the X-chromosome, XIST induces repressive chromatin changes and gene expression dampening at its autosomal targets (Dror et al, Cell, 2024). Thus, XIST equalizes X-linked gene dosage between male and female cells while inducing differences on autosomes. Intriguingly, we also found this dispersed Xist localization and autosomal binding in a brief window during the initiation of X-chromosome inactivation in the mouse system (Dror et al, Cell, 2024), indicating that the autosomal function of Xist is conserved.
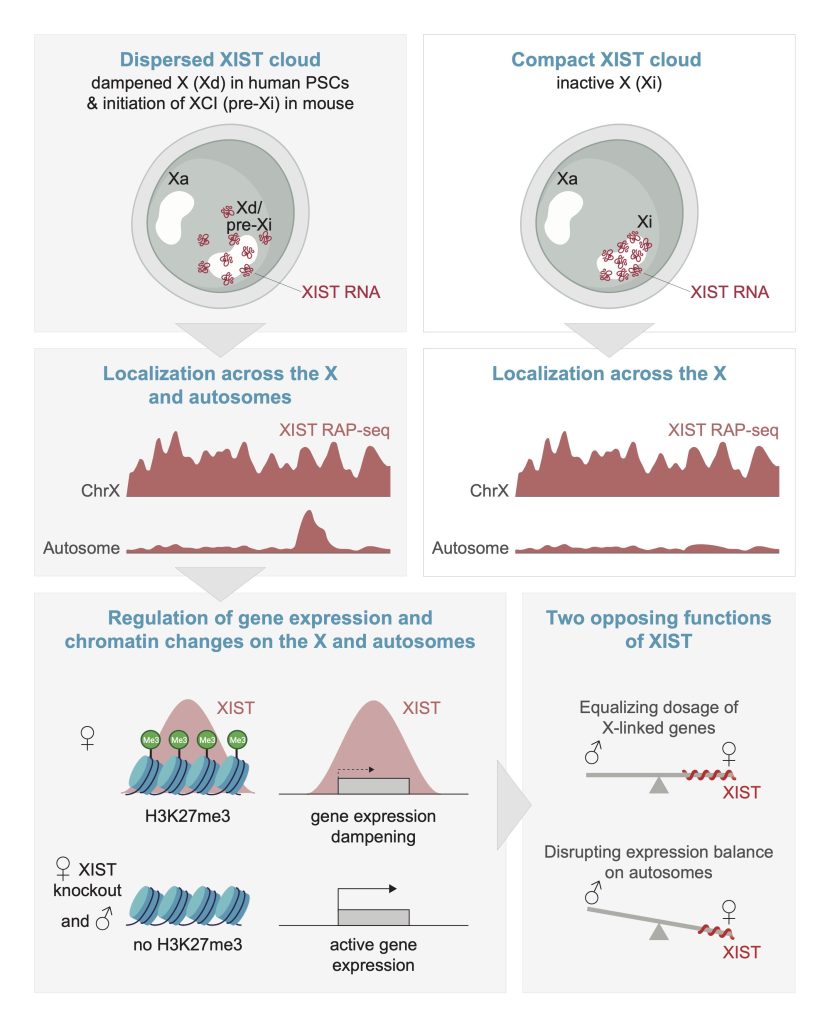
Our work establishes a new role for XIST/Xist and shifts the paradigm from XIST/Xist being a cis-limited regulator to showing that it can also operate in trans. We suggest that autosomal regulation by XIST/Xist needs to be considered in cell types with a dispersed distribution of the RNA, which for example has been reported in immune and cancer cells. In immune cells, this autosomal action of XST might contribute to the female bias of autoimmune diseases. This is an exciting new perspective in XIST biology!
Inactive X-chromosome erosion in primed human pluripotent stem cells
The X-chromosome status of primed human pluripotent stem cells is complex. At earliest passage, female primed human pluripotent stem cells (hPSCs, including both embryonic stem cells and induced pluripotent stem cells) carry an inactive X chromosome expressing and coated with XIST RNA and one active X chromosome (Patel et al, Cell Reports, 2016, Tchieu et al, Cell Stem Cell, 2010). Over time in culture (~passage 5-15 after derivation of the lines), they lose XIST expression due to silencing by DNA methylation and a large subset of genes on the inactive X reactivates, resulting in higher dosage of these genes. The dysregulation of the Xi in female hPSCs is coined Xi-erosion and the deregulated inactive X chromosome, the eroded X (Xe). We showed that a defining feature of Xi erosion is that it cannot be undone, even during differentiation (Patel et al, Cell Reports, 2016). In other words, the aberrant X chromosome state of female hPSC lines is locked in place so that, upon differentiation, the re-activated parts of the Xe cannot be re-silenced, resulting in differentiated cells with an increased dose of eroded X-linked genes. Thus, downstream applications of primed human pluripotent stem cells (which is the state of pluripotent cells used by most labs) are adversely affected by the lack of proper X-chromosome dosage compensation. Intriguingly, we showed that the conversion to the naïve pluripotent state can erase the epigenetic abnormalities of the X found in primed pluripotent stem cells and allow for proper XCI upon differentiation (Sahakyan et al, Cell Stem Cell, 2016).
Current directions in the lab
Overall, our research underscores that there is still much more to uncover about the X chromosome and Xist RNA, and we are committed to further exploration.
- Can we build a complete map of all the regulatory elements of XIST?
- Can we understand why X-chromosome compensation matters for development and homeostasis?
- Can we understand the mechanisms that distinguish XCI and XCD?
- Can we understand the mechanisms that control the escape from X-chromosome inactivation?
- Can we define the functional relevance of autosomal Xist binding for development, homeostasis, and disease? How does this new function of Xist alter female biology in comparison to that of males?
- Can we define the structure and assembly of Xist-SMACs?
- Can we define how XIST expression and escape from X-chromosome inactivation in females alters cellular functions and leads to female-biased diseases such as autoimmunity?
- Can we define how the deregulation of XIST and X-chromosome inactivation leads to cancer?